Future 5G cellular networks
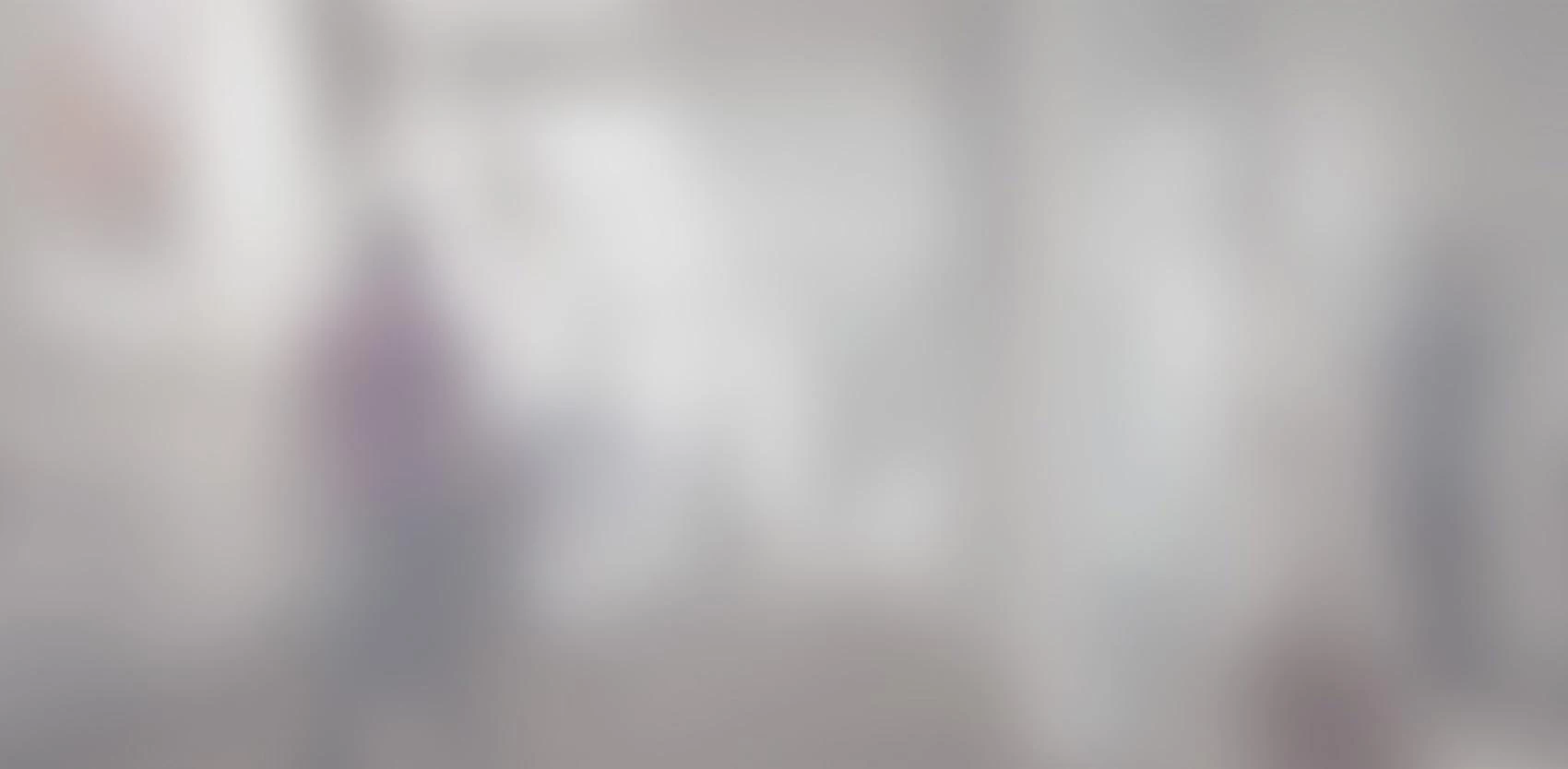
Since the introduction of the General Packet Radio Service (GPRS) standard derived from GSM in 2000, consumers and telecom companies have been demanding ever higher transfer speeds on mobile networks.
Since the introduction of the General Packet Radio Service (GPRS) standard derived from GSM in 2000, consumers and telecom companies have been demanding ever higher transfer speeds on mobile networks. It is true that increasing the data transfer speed of cellular networks would be an asset for our M2M projects. Who could really complain about it? It is fair to say that the desire to continually increase transfer speeds on cellular communications networks is one of the greatest motivations behind the research underway to define and design 5G. While the deployment of 5G networks is not for tomorrow (we are talking about 2020), engineers have already started researching the technologies that will make up 5G, hoping to achieve much faster speeds than today. When we look at the evolution of GPRS (commonly called 2.5G) to Long Term Evolution Advanced (LTE-Advanced or 4G), the recipe to produce higher transfer speeds seems relatively simple. However, it will take several more years of research before an official definition for 5G is reached. In fact, the combination of higher bandwidth channels (from 200 kHz to 100 MHz), higher-order modulation regimes from Gaussian Minimum Shift Keying (GMSK) to 64-state Quadruple Amplitude Modulation (64QAM) and Multiple-Input Multiple-Output (MIMO) technology enables modern cellular networks, such as LTE-Advanced, to reach transfer speeds much higher than the good old GPRS, allowing to go from 56 kbits/s to 3 Gbits/s. Wow!
The evolution of network architecture
While it is clear that 5G cellular networks will incorporate a new physical layer, the use of multiple inputs, massive multiple outputs and millimeter radio waves, it is important to understand these innovations from a network architecture evolution perspective. Historically, a cellular network consisted of a single base station serving a large number of users. Today, however, the modern architecture of cellular networks is becoming more and more heterogeneous, for good reasons. In these heterogeneous networks, also known as "HetNets", base stations are often augmented with a large number of small cells such as femtocells and picocells. These small cells are actually miniature base stations that can be used to improve coverage in harsh environments and increase network capacity. All indications are that, in the medium to long term, cellular networks will use an increasing number of small cells. In addition, telecommunication-class Wi-Fi radios can definitely contribute to cellular networks by increasing capacity. In fact, these evolutions in network topology are already underway. Engineers can now consider technologies that were considered utopian in the past for present and future cellular communications. For example, engineers can now, thanks to the reduction in cell size, consider using a higher frequency range that was not viable, at the time, because of propagation problems over distance.
New physical layers
The first area of research in 5G cellular networks is, without a doubt, the evolution of the physical layer. Historically, the evolution of the physical layer had allowed a higher transfer speed thanks to the use of higher order modulation and a more sophisticated signal structure. For example, the progression from GSM/EDGE to Universal Mobile Telecommunications System (UMTS) introduced Code Division Multiple Access (CDMA) technology. In addition, the progression of LTE had involved the introduction of Orthogonal Frequency Division Multiplexer (OFDM) and Single-Carrier Frequency Division Multiple Access (SC-FDMA). As then, researchers continue to design and make signal structures more efficient. For example, Generalized Frequency Division Multiplexing (GFDM) uses a special filter to mitigate adjacent channel interference compared to traditional OFDM. In addition, technologies such as the Non-Orthogonal Multiple Access Multiplexer (NOMA) use the transmission energy domain for better spectrum utilization. GFDM and NOMA are just two of the areas to be explored for new signal structures that will be designed to increase capacity and efficiency within a given spectrum.
Multiple Inputs, Multiple Outputs (MIMO) Ground
MIMO technology enables faster and longer distance data transfer across cellular networks compared to SISO technology (single input, single output). MIMO is the second research area for 5G. Standard Global System for Mobile Communications (GSM) networks use a single antenna at the receiver and transmitter, while MIMO technology, on the other hand, uses multiple antennas at both the receiver and transmitter. Over the past decade, MIMO technology has opened the door to increasing capacity per channel beyond what the Shannon-Hartley theorem theoretically allowed. The primary premise of MIMO is that the use of multiple antennas allows data transmission over a larger spectrum. The use of multiple antennas allows the transmission of data streams to be split and improves the quality of the signal-to-noise ratio. Nowadays, several cellular communication standards allow the use of MIMO to improve capacity per channel. Since 2007 with the delivery 7 of the 3rd Generation Partnership Project (3GPP), the Evolved High Speed Packet Access (HSPA+) already supported downstream transmission with a 2 by 2 MIMO. In addition, the LTE standard introduced with 3GPP delivery 8 in 2008 allowed for an 8-by-8 MIMO. The use of MIMO technology in our wireless networks, even today, has continued to grow. This leads us to believe that the next generations of cellular communication networks will most likely use MIMO systems with a much higher number of antennas. We will therefore speak of multiple inputs, multiple mass outputs. Researchers at Lund University in Sweden are using Software Defined Radio (SDR) systems to prototype a concept of multiple-input, multiple-output ground communication links (see LUND University Massive MIMO). In addition to using multiple antennas to allow a higher data transfer rate, the system will focus energy for beamforming to a specific wireless device.
Millimeter radio waves
Not surprisingly, we can announce that millimeter radio waves are the third area of research. The majority of today's cellular networks operate in particularly narrow bands below 2 GHz, where signals travel long distances in the open air, but remain in a somewhat limited spectrum. The bandwidth of the available spectrum affects the maximum speed of data transmission in these bands. According to Shannon-Hartley's theorem, capacity is a linear function of bandwidth according to the following formula: C = B log2 (1 + S / N) Where: C is the channel capacity in bits per second B is the channel bandwidth in hertz S is the received signal power over the bandwidth in watts and N is the average noise over the bandwidth in watts. Given the limitations of the spectrum below 2 GHz, researchers are exploring frequencies that can support greater capacity. Among the most promising bands for future cellular networks are millimeter radio waves such as 28 Ghz, 38 Ghz and 72 GHz. Millimeter waves or Extremely High Frequency (EHF) are the frequencies that lie between 1 millimeter and 1 centimeter, i.e. from 30 to 300 GHz. Millimeter waves offer a bandwidth that is substantially greater than the bands currently used in telecommunications.
Conclusion
We are already preparing for the arrival of 5G. The fields of machine-to-machine (M2M) and connected objects (IoT) will be able to make good use of this highly anticipated technological evolution.